Sørensen Group - Renal Hemodynamics
Each day the kidney filters 180 L of fluid and more than 500 g of sodium. The balance between how much salt and water is filtered and how much is excreted in the urine has a huge impact on your blood pressure.
The kidney regulates salt and water excretion and the kidney function is crucial in the regulation of blood pressure. In the western world high blood pressure (hypertension) is a major health problem which has fatal consequences such as renal failure, stroke and myocardial infarction. The mechanisms behind the development and maintenance of hypertension are largely unknown despite intense research.
We suggest that changes in kidney function are involved in the development of hypertension.
Our aim is to understand how the renal vasculature functions during normal conditions and during disease-states such as hypertension and diabetes. We investigate the mechanisms regulating the excretory function of the kidney. This includes control of renal blood flow (RBF), glomerular filtration rate (GFR) and the renin-angiotensin system. We focus on the structure of the vascular tree, intercellular communication via gap junctions, vascular regulation via ion channels, the sympathetic nervous system and changes in renal autoregulation.
In vivo methods
Renal function studies
We measure renal blood flow, glomerular filtration, blood pressure, heart rate and urine excretion under different experimental conditions:
- Changes in blood pressure
- Infusion of pharmacological substances
a. Into the blood stream
b. Directly into the kidney - Treatment over longer periods (chronic)
Super Resolution Imaging
We scan the kidneys of anesthetized rats using microbubbles and ultrasound (contrast enhanced ultra sound) to generate images of the renal vasculature and measurements of flow in different kidney regions in healthy and ischemic kidneys.
Genetically modified animals
We use different disease model e.g. hypertensive or diabetic rats to probe the differences in renal function. We use different genetically modified models e.g. knock-out mice lacking expression of different connexins, ion channels (K+, Ca2+ etc.), receptors (GLP-1, glucagon etc) to monitor changes in renal hemodynamics.
Telemetry
We measure blood pressure and ECG in awake animals using telemetry during different treatment regimes and in the different models mentioned above.
In vitro methods
Isolated arterioles mounted in wire-myographs or pressure myographs are used for Ca2+ measurements, vascular responses to agonists/antagonists, myogenic responses and flow-induced diameter changes. Vessels are obtained from healthy animals, different disease models or genetically modified animal models.
Isolated perfused rat or mouse kidneys are used to measure changes in the diameter of afferent or efferent arterioles. Both local and propagated vascular responses can be measured during:
- Changes in perfusion pressure
- Changes in tubular flow
- Pharmacological intervention
- Electrical stimulation
The renal vascular tree displays a highly irregular branching pattern such that the pre-glomerular resistance may differ markedly between two adjacent glomeruli. In spite of this, the filtration pressure is remarkably similar in different glomeruli.
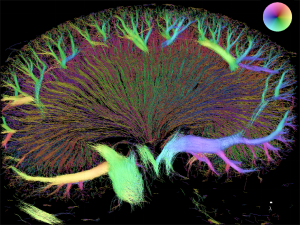
Each organ has specific requirements for the vascular tree perfusing it. The kidney receives app. 1L of blood per min (1/5 of cardiac output) and filters 180L of plasma every day. This requires a high filtration pressure and a tight regulation of flow and pressure. In the kidneys, this control is driven in part by the ability of the vascular cells to communicate through gap junction. The structure of the renal vascular wall and the renal vascular tree are uniquely suited for this. The challenge in research is to generate a full 3D structure of the renal vascular tree in vivo. We will accomplish this using super-resolution imaging (SRI). Ultrasound scans of kidneys perfused with micro-bubbles will reveal the unique structure of the renal vascular tree in vivo. With this technique, we aim to estimating flow and pressure within the renal vasculature. Comparing kidneys from healthy and diseased (hypertensive, diabetic) animals will answer many questions regarding development of renal diseases.
A further advancement is the use of red blood cells as tracking target (substituting microbubbles) to convert super-resolution ultrasound into a non-invasive method. This high-gain high-risk project is funded by a Synergy Grant from the European Research Council (ERC).
The projects are a collaboration between the Sørensen group and researchers at DTU and Rigshospitalet.
Renal effects of GLP-1 and glucagon
The gut hormone GLP-1 also has renal effects. It increases renal blood flow, glomerular filtration rate and sodium excretion. We have shown that GLP-1 receptors are expressed in renal vascular smooth muscle cells but expression is significantly reduced in hypertensive animals. GLP-1 also attenuates renal autoregulation. This implicates GLP-1 as a major contributor in the control of renal hemodynamics. Furthermore, GLP-1 agonists has been shown to protect kidney function in patients with renal failure.
Glucagon increases renal excretion of sodium and potassium. It is known, that plasma levels of glucagon are high in people with type-2 diabetes. We are investigating the potential damaging effects of high plasma levels of glucagon on kidney function and a possible role in development of renal failure.
Ongoing GLP-1 projects
GLP-1 and sympathetic activity
Interactions between the sympathetic nervous system and GLP-1 in in the control of renal hemodynamics. Investigations in whole animals to assess the effect of GLP-1 on renal autoregulation and sodium excretion.
GLP-1 receptors in human kidneys
We are exploring GLP-1 receptor expression this using human renal tissue from healthy, diabetic and hypertensive patients to examine the location and possible changes in expression during diseases. Collaboration with SDU.
GLP-1 in renal vascular responses
GLP-1 induces renal vasodilation but the pathway is unclear. In isolated renal vessels we examine changes in intracellular Ca2+ and the effect of different ion channels inhibitors and activators.
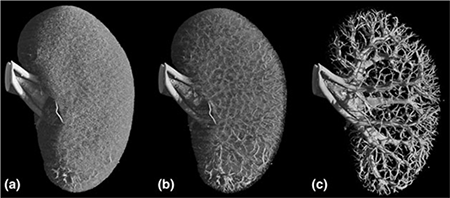
Gap junctions allow signals to travel between cells. In the renal vasculature, gap junctions are found between vascular smooth muscle cells, between endothelial cells and between vascular smooth muscle cells - endothelial cells. Stimulation in one part of an arteriole leads to a response at a distant site. We have shown that this signal conduction participates in renal autoregulation. Also, the control of the renin-angiotensin system depends on functional gap junctions. This implicates gap junctions as a major contributor in the control of renal hemodynamics.
The myogenic response and the tubuloglomerular feedback (TGF) autoregulate renal blood flow and glomerular filtration rate. This depends on a functional communication between the vascular cells. Renal gap junctions are built from different connexin (Cx) isoforms: Cx37, Cx40, Cx43 and Cx45. The function and expression of Cx is changed during hypertension and diabetes.
Ongoing gap junction projects
In cooperation with Associate Professor Jens Christian Brings Jacobsen these experimental data is used to generate mathematical models of the renal autoregulation in order to elucidate the changes occurring in the vascular wall when intercellular communication is reduced.
In collaboration with Associate Professor Olga Sosnovtseva we investigate the changes in TGF induced by different gap junction upcouplers. We also investigate how changes in tubular flow (induced e.g. by SGLT2 inhibitors) affect renal autoregulation.
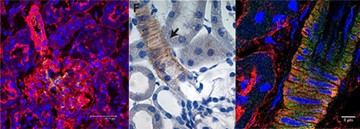
Ion channels in the renal resistance vessels affect the cell membrane potential. This leads to changed permeability of voltage sensitive Ca2+ channels and vascular tone. We have shown that in diet induced and genetically induced hypertension the expression and function of vascular K+ channels is changed. This implicates K+ channels as a major contributor in the control of renal hemodynamics.
Ongoing ion channel projects
The vasodilatory effects of GLP-1 and glucagon may be regulated through K+ channels. This is explored in isolated renal arteries and in whole animals.
Partners
![]() |
Jørgen Arendt Jensen |
![]() |
Erik Villain Thomsen |
![]() |
Michael Bachmann Nielsen |
![]() |
Professor Will Couples |
![]() |
Associate professor Lisa Harrison-Bernard Louisiana State University USA |
![]() |
Professor Donald Marsh, University of Southern California USA |
![]() |
Associate professor Lars Jørn Jensen Science, University of Copenhagen Denmark |
![]() |
Associate Professor Brant Isakson University of Virginia USA |
Funding
2021: Novo Nordisk Foundation. 2.834.660 DDK for the project “Renal effects of glucagon and the association to development and treatment of chronic kidney disease in diabetes”.
2020 + 2021: Augustinus Fonden. 2.000.000 DDK for research in the role of glucagon in development of diabetic kidney disease.
2019: European Research Council (ERC) Synergy Grant. 10.000.000 Euro – Super resolution Ultrasound imaging of Erythrcytes (SURE) in the healthy and the diabetic kidney.
2018: Novo Nordisk Foundation. 8.000.000 DDK for a research infrastructure examining the cardiovascular phenotypes in rodent models. You can read more about the infrastructure the Telemetry Unit at our website.
2017: Innovation Fund Denmark. 1.458.000 DDK for Super Resolution Imaging (SRI) of the kidney.
2017: Innovation Fund Denmark. 450.000 DDK for Translational models of diabetic nephropathy.
Over the years the lab has received other funding from (alphabetic order):
- A.P. Møller
- Aase & Ejnar Danielsens Foundation
- Helen and Ejnar Bjørnows Foundation
- König-Petersen
- The Danish Heart Foundation
- The Lundbeck Foundation
Group Leader
Charlotte Mehlin Sørensen
Associate Professor
Telephone: +4528757404
E-mail: cmehlin@sund.ku.dk
ORCID: 0000-0002-9984-3125
Group members
Name | Title | Phone | |
---|---|---|---|
Amy McDermott | PhD Fellow | +4535323862 | |
Anna Billeschou Bomholt | PhD Fellow | ||
Christian Aalkjær | Emeritus | +4535334235 | |
Emma Tina Bisgaard Olesen | Associate Professor | ||
Karin Larsen | Laboratory Technician | +4535324061 | |
Nathalie Sarup Panduro | Medical student | ||
Thomas Jonassen | Associate Professor | +4535327616 | |
Vishalini Venkatesan | PhD Fellow | +4535325621 |